El sistema de escape de la tripulacion del F 111 es muy particular, acá va el artículo completo sobre el mismo ( en inglés). Hacer click acá para ver otros sistemas de escape
Chapter 1 Escape and Survival Systems Description
219. System overview
220. Pre-ejection
221. Ejection
222. Post ejection (recovery system)
223. Stabilization system
Chapter 2 Crew Module Ejection Sequence
224. Ejection sequence
225. Time-Delay Initiation
The development of the high-speed F-111 aircraft caused the need for an improved egress system. The ejectable crew module was designed to meet this need. The system provides maximum protection for the crewmembers throughout the aircraft performance envelope and includes capabilities for safe ejections at maximum speed and altitude as well as at zero altitude and 50 knots indicated airspeed (KIAS). The module is self-righting, watertight, has flotation provisions, and provides protection for the crewmembers from environmental hazards met on land or water. A side-by-side crew arrangement facilitates safe and effective performance and allows crewmembers to work together and aid one another in performing mission tasks while still maintaining their forward visibility which is an important factor in high-speed, low-level flights.
In this unit we will look at the many systems that make up the crew module. We will break these systems down into components, tie these components together and trace the ejection sequence.
Chapter 1. Escape and Survival Systems Description
The crew module is composed of many systems such as:
Initiation.
Severance.
Separation.
Stabilization.
Recovery.
Landing.
Flotation.
Survival.
Seat and restraint.
Emergency oxygen.
They are interconnected by means of shielded mild detonating cord (SMDC) which acts as a stimulus transfer medium. The SMDC is provided with time delay initiators (TDI) and one-way explosive transfers to ensure proper sequencing of the various functions. Explosive transfer connectors are incorporated in the system for firing redundancy. After actuation of the initiation system, sequencing of all systems through landing and flotation is automatic. The escape and survival systems consist of the crew module system and oxygen systems. The oxygen systems are the normal (liquid) oxygen system, oxygen quantity and pressure warning system, and emergency (gaseous) oxygen system.
219. System overview Now that you have a general idea of what makes up the crew module ejection system, let's take a closer look at the module system and the oxygen system. Let's begin with the crew module.
Crew module. The crew module, (fig. 1 ), provides maximum comfort and protection for both crewmembers during normal and emergency conditions. It is integrated into the F-111 aircraft encompassing the pressurized cabin and forward portion of the wing glove. The two crew seats are positioned side by side and have restraints incorporated that enhance freedom of movement and comfort by eliminating the need for a personal parachute and survival equipment to be fitted to the crewmembers. Survival equipment and a recovery parachute are a part of the crew module system. During an emergency, the crew module is separated from the aircraft and propelled to a height sufficient for successful recovery throughout the aircraft performance envelope. The system has features to reduce the landing shock on land or water and has self-righting buoyancy, flotation capacity, and protects the crewmembers against environmental hazards.
Oxygen system. The three functions of the oxygen systems are normal, quantity and pressure warning, and emergency. During normal operations, the normal (liquid) oxygen system supplies the crewmembers with breathing oxygen. When the normal oxygen system malfunctions, low system pressure and low liquid oxygen quantities are indicated by a normal oxygen quantity and pressure warning system.
Figure 1. F-111 crew module.
An emergency (gaseous) oxygen system supplies breathing oxygen to crewmembers during normal oxygen system failure or during an ejection.
220. Pre-ejection
As stated earlier, the crew module escape system is made up of many systems which work together during an ejection. These systems can be divided into three categories: pre-ejection, ejection, and post-ejection. The pre-ejection category consists of the ejection initiators, guillotines, emergency oxygen, mechanical explosive interrupt, a radio beacon, a 3.0-second TDI, chaff, and a .35-second TDI. Let's take a closer look at some of the components that may be unfamiliar to you.
Ejection initiators. The ejection initiators are used to start the sequence to separate and eject the crew module from a disabled aircraft, much like the ejection initiators used in any other aircraft. However, as you can see in figure 2, the initiators used in the F-111 are quite different in looks and operation. Safety pins are normally installed in the ejection initiator handles to prevent accidental firing of the initiators, whenever aircraft maintenance is being performed.
An ejection initiator on each side of the center console is within easy reach of both crewmembers and permits either crewmember to start the crew module ejection sequence. Each initiator has a D-shaped grip that must be squeezed and then pulled upward to actuate the built-in firing mechanism. Squeezing the grip releases the initiator's built-in lock release. As the grip is pulled, the firing mechanism first compresses the firing pin springs. As the grip reaches its upper limits, the spring tension drives the firing pins into a percussion-type primer. The primer actuates the explosive train and the explosive crossover.
Figure 2. Ejection initiator.
Guillotines. Guillotines sever antenna leads, secondary control cables, and an oxygen line. Refer to the guillotines in figure 3. The cartridge is fired by the SMDC to actuate the guillotine blade for severance. The secondary controls guillotine is located on the bottom surface of the crew module floor in the left cheek area of the fuselage, the blade antenna leads guillotine is located on the centerline of the crew module glove beneath access cover 2410, and the leading edge antenna leads guillotine is located on the centerline of the crew module glove beneath access cover 2420.
Emergency oxygen. The emergency oxygen system provides both crewmembers with a 10-minute supply of oxygen during ejection or when the normal aircraft oxygen system fails. During the pre-ejection sequence, the emergency oxygen system is actuated explosively by the SMDC from the ejection initiators. If the normal oxygen system fails during flight, the emergency system can be turned on manually.
Mechanical explosive interrupt (MEI). The MEI is a unit, that is controlled by the crewmember with a chaff interrupt lever, that allows or stops the explosive propagation to the emergency radio beacon and the 3.0-second TDI which, when fired, actuates the chaff dispenser. If the unit is closed, then propagation is stopped. The TDI and emergency radio beacon are not activated. If the unit is open, then propagation continues, activating both the emergency radio beacon and a 3-second TDI. The TDI gives the crew module time to clear the aircraft before it fires, actuating the chaff dispenser.
Radio beacon. The radio beacon sends out radio signals for rescue purposes after an ejection. If the MEI is in the open position, the beacon will automatically send out its signal. If the MEI is closed, the radio beacon can be manually operated by a switch in the cockpit.
.35-second TDI. The .35-second time-delay initiator is fired by the SMDC from the ejection initiators and delays the actuation of the components that make up the severance system.
221. Ejection
Now let's look at the ejection category which consists of flexible linear-shaped charges (FLSC), two. 15-second time-delay initiators, a rocket motor, a dualmode, q-actuated selector, a 1.6-second time-delay initiator, a 4.4-second timedelay initiator, a 1.0-second time-delay initiator, a "G" sensor initiator, and the select/interrupt valve.
Flexible linear-shaped charge. FLSC severs the module from the aircraft. It is installed around certain covers and splice plates on the module to cut the metal for severance and is formed in a chevron-shaped cross section for use in severance strips. Also, it is used to cut a larger hole in the upper nozzle of the rocket motor during high-speed ejections. A booster tip is installed on each end. The amount of explosive per foot of FLSC is selected to cut a specified thickness of metal.
.15-second TDI. Them are two .15-second time-delay initiators installed in the ejection system. One delays firing of the stabilization/brake parachute catapult until the module has separated from the aircraft and the other delays firing of the FLSC in the rocket motor upper nozzle in mode 1 until the module has cleared the aircraft.
Rocket motor. In figure 4, you see that the rocket motor is composed of an upper closure or compartment, a 9-inch by 58-inch steel cylinder case, and a lower closure or compartment. Starting at the top of the upper closure, the SMDC propagation actuates the firing pin. This in turn fires the percussion primer in the igniter cup, which then detonates the motor ignition pellets. These ignition pellets are suspended in a foam that aids in their detonation. The rapidly detonating ignition pellets cause the propellant grain in the steel cylinder to be ignited.
Figure 3. Rocket motor.
The rocket motor lower nozzle provides 27,000 pounds of thrust. To avoid excessive "g" forces to the crewmembers, the rocket motor is provided with two concentric upper nozzles, secondary and auxiliary. The small auxiliary nozzle in the center of the upper nozzle fires simultaneously with the lower nozzle. This action provides 500 pounds of thrust to counteract slow-speed crew module pitch up at speeds below 300 knots. At speeds above 300 knots, after a .15-second delay, the upper nozzle burst diaphragm is severed by a flexible linear-shaped charge (FLSC) to increase the exhaust-flow area, thus increasing its thrust. Because of the increase in the exhaust-flow area, the rocket motor operating pressure is lowered, which results in reduced thrust of 9,000 pounds at the lower nozzle and increases the upper nozzle thrust to 7,000 pounds. This overall decreased thrust extends the operating time and reduces excessive "g" forces.
Dual-mode, q-actuated selector. In figure 5, you see the dual-mode, q-actuated selector. It continuously senses aircraft speed and selects the appropriate time delay. The letter "q" is used to identify forces or pressure required to actuate various pressure-sensitive aircraft devices. Pressure from the pitot static system, or ram pressure, is sensed at one end of the q-actuated selector, while dynamic pressure is sensed at the other end. Due to differential pressure, the q-actuated selector allows activation of a 1-second TDI to the barostat lock initiator and blocks propagation to the rocket motor upper nozzle when aircraft speed is less than 300 knots. When aircraft speed is greater than 300 knots, differential pressure is changed so that the q-actuated selector blocks propagation to the 1-second TDt and allows activation of SMDC to the. 15-second TDI to the rocket motor upper nozzle to fire.
4.4-second TDI. Another explosive train, with a 4.4-second time-delay initiator, is provided to back up both the dual-mode, qactuated selector and the g-sensor initiator. This equipment ensures that the barostat lock initiator is never activated more than 4.4 seconds after the rocket motor is fired.
1.0-second TDI. This time delay allows the module to gain altitude during a mode 1 ejection. Upon actuation, it fires the barostat lock initiator.
G-sensor initiator. The g-sensor initiator (fig. 6) is located in the survival equipment explosive device compartment. A TDI delays firing the g-sensor initiator for 1.6 seconds after rocket motor ignition during high-speed ejections. The forward motion of the crew module may be relatively high immediately after ejection of the crew module at speeds above 300 knots. After the forward motion decreases to approximately 2.2 (+/- 1 ) g' s, the g-sensor initiator fires and activates the barostat lock initiator.
Select/interrupt valve. The select/interrupt valve works similarly to the mechanical explosive interrupt. It either allows or blocks explosive propagation leading to the stabilization/brake parachute cutters. The direction of propagation depends on the mode of ejection selected by the dual-mode, q-actuated selector. When a mode 1 is selected, a detonation transfer assembly (DTA), which is another type of mild detonating cord, repositions the select/interrupt valve. When the recovery system operates, DTA propagation passes through the repositioned valve and fires the cutters.
Chapter 1 Escape and Survival Systems Description
219. System overview
220. Pre-ejection
221. Ejection
222. Post ejection (recovery system)
223. Stabilization system
Chapter 2 Crew Module Ejection Sequence
224. Ejection sequence
225. Time-Delay Initiation
The development of the high-speed F-111 aircraft caused the need for an improved egress system. The ejectable crew module was designed to meet this need. The system provides maximum protection for the crewmembers throughout the aircraft performance envelope and includes capabilities for safe ejections at maximum speed and altitude as well as at zero altitude and 50 knots indicated airspeed (KIAS). The module is self-righting, watertight, has flotation provisions, and provides protection for the crewmembers from environmental hazards met on land or water. A side-by-side crew arrangement facilitates safe and effective performance and allows crewmembers to work together and aid one another in performing mission tasks while still maintaining their forward visibility which is an important factor in high-speed, low-level flights.
In this unit we will look at the many systems that make up the crew module. We will break these systems down into components, tie these components together and trace the ejection sequence.
Chapter 1. Escape and Survival Systems Description
The crew module is composed of many systems such as:
Initiation.
Severance.
Separation.
Stabilization.
Recovery.
Landing.
Flotation.
Survival.
Seat and restraint.
Emergency oxygen.
They are interconnected by means of shielded mild detonating cord (SMDC) which acts as a stimulus transfer medium. The SMDC is provided with time delay initiators (TDI) and one-way explosive transfers to ensure proper sequencing of the various functions. Explosive transfer connectors are incorporated in the system for firing redundancy. After actuation of the initiation system, sequencing of all systems through landing and flotation is automatic. The escape and survival systems consist of the crew module system and oxygen systems. The oxygen systems are the normal (liquid) oxygen system, oxygen quantity and pressure warning system, and emergency (gaseous) oxygen system.
219. System overview Now that you have a general idea of what makes up the crew module ejection system, let's take a closer look at the module system and the oxygen system. Let's begin with the crew module.
Crew module. The crew module, (fig. 1 ), provides maximum comfort and protection for both crewmembers during normal and emergency conditions. It is integrated into the F-111 aircraft encompassing the pressurized cabin and forward portion of the wing glove. The two crew seats are positioned side by side and have restraints incorporated that enhance freedom of movement and comfort by eliminating the need for a personal parachute and survival equipment to be fitted to the crewmembers. Survival equipment and a recovery parachute are a part of the crew module system. During an emergency, the crew module is separated from the aircraft and propelled to a height sufficient for successful recovery throughout the aircraft performance envelope. The system has features to reduce the landing shock on land or water and has self-righting buoyancy, flotation capacity, and protects the crewmembers against environmental hazards.
Oxygen system. The three functions of the oxygen systems are normal, quantity and pressure warning, and emergency. During normal operations, the normal (liquid) oxygen system supplies the crewmembers with breathing oxygen. When the normal oxygen system malfunctions, low system pressure and low liquid oxygen quantities are indicated by a normal oxygen quantity and pressure warning system.
Figure 1. F-111 crew module.
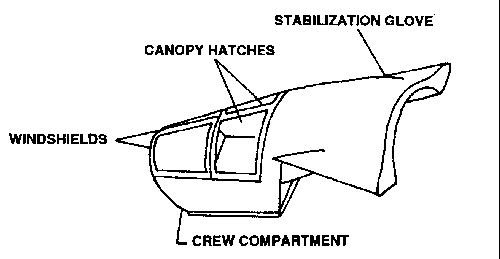
An emergency (gaseous) oxygen system supplies breathing oxygen to crewmembers during normal oxygen system failure or during an ejection.
220. Pre-ejection
As stated earlier, the crew module escape system is made up of many systems which work together during an ejection. These systems can be divided into three categories: pre-ejection, ejection, and post-ejection. The pre-ejection category consists of the ejection initiators, guillotines, emergency oxygen, mechanical explosive interrupt, a radio beacon, a 3.0-second TDI, chaff, and a .35-second TDI. Let's take a closer look at some of the components that may be unfamiliar to you.
Ejection initiators. The ejection initiators are used to start the sequence to separate and eject the crew module from a disabled aircraft, much like the ejection initiators used in any other aircraft. However, as you can see in figure 2, the initiators used in the F-111 are quite different in looks and operation. Safety pins are normally installed in the ejection initiator handles to prevent accidental firing of the initiators, whenever aircraft maintenance is being performed.
An ejection initiator on each side of the center console is within easy reach of both crewmembers and permits either crewmember to start the crew module ejection sequence. Each initiator has a D-shaped grip that must be squeezed and then pulled upward to actuate the built-in firing mechanism. Squeezing the grip releases the initiator's built-in lock release. As the grip is pulled, the firing mechanism first compresses the firing pin springs. As the grip reaches its upper limits, the spring tension drives the firing pins into a percussion-type primer. The primer actuates the explosive train and the explosive crossover.
Figure 2. Ejection initiator.
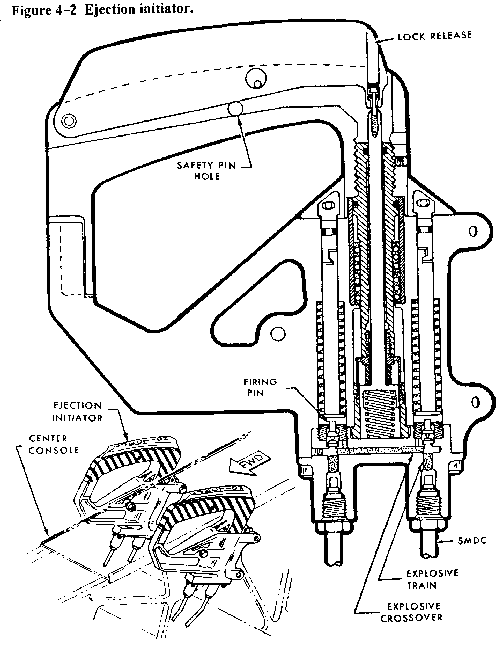
Guillotines. Guillotines sever antenna leads, secondary control cables, and an oxygen line. Refer to the guillotines in figure 3. The cartridge is fired by the SMDC to actuate the guillotine blade for severance. The secondary controls guillotine is located on the bottom surface of the crew module floor in the left cheek area of the fuselage, the blade antenna leads guillotine is located on the centerline of the crew module glove beneath access cover 2410, and the leading edge antenna leads guillotine is located on the centerline of the crew module glove beneath access cover 2420.
Emergency oxygen. The emergency oxygen system provides both crewmembers with a 10-minute supply of oxygen during ejection or when the normal aircraft oxygen system fails. During the pre-ejection sequence, the emergency oxygen system is actuated explosively by the SMDC from the ejection initiators. If the normal oxygen system fails during flight, the emergency system can be turned on manually.
Mechanical explosive interrupt (MEI). The MEI is a unit, that is controlled by the crewmember with a chaff interrupt lever, that allows or stops the explosive propagation to the emergency radio beacon and the 3.0-second TDI which, when fired, actuates the chaff dispenser. If the unit is closed, then propagation is stopped. The TDI and emergency radio beacon are not activated. If the unit is open, then propagation continues, activating both the emergency radio beacon and a 3-second TDI. The TDI gives the crew module time to clear the aircraft before it fires, actuating the chaff dispenser.
Radio beacon. The radio beacon sends out radio signals for rescue purposes after an ejection. If the MEI is in the open position, the beacon will automatically send out its signal. If the MEI is closed, the radio beacon can be manually operated by a switch in the cockpit.
.35-second TDI. The .35-second time-delay initiator is fired by the SMDC from the ejection initiators and delays the actuation of the components that make up the severance system.
221. Ejection
Now let's look at the ejection category which consists of flexible linear-shaped charges (FLSC), two. 15-second time-delay initiators, a rocket motor, a dualmode, q-actuated selector, a 1.6-second time-delay initiator, a 4.4-second timedelay initiator, a 1.0-second time-delay initiator, a "G" sensor initiator, and the select/interrupt valve.
Flexible linear-shaped charge. FLSC severs the module from the aircraft. It is installed around certain covers and splice plates on the module to cut the metal for severance and is formed in a chevron-shaped cross section for use in severance strips. Also, it is used to cut a larger hole in the upper nozzle of the rocket motor during high-speed ejections. A booster tip is installed on each end. The amount of explosive per foot of FLSC is selected to cut a specified thickness of metal.
.15-second TDI. Them are two .15-second time-delay initiators installed in the ejection system. One delays firing of the stabilization/brake parachute catapult until the module has separated from the aircraft and the other delays firing of the FLSC in the rocket motor upper nozzle in mode 1 until the module has cleared the aircraft.
Rocket motor. In figure 4, you see that the rocket motor is composed of an upper closure or compartment, a 9-inch by 58-inch steel cylinder case, and a lower closure or compartment. Starting at the top of the upper closure, the SMDC propagation actuates the firing pin. This in turn fires the percussion primer in the igniter cup, which then detonates the motor ignition pellets. These ignition pellets are suspended in a foam that aids in their detonation. The rapidly detonating ignition pellets cause the propellant grain in the steel cylinder to be ignited.
Figure 3. Rocket motor.
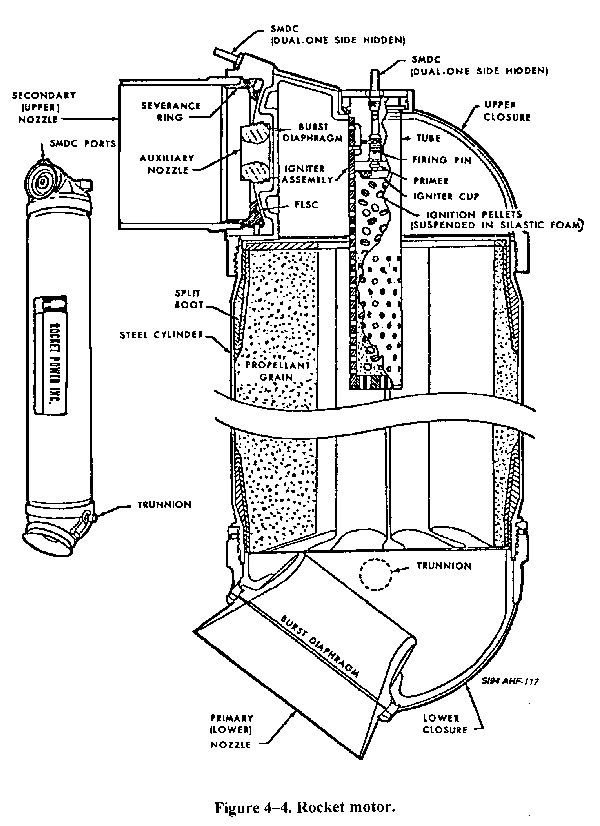
The rocket motor lower nozzle provides 27,000 pounds of thrust. To avoid excessive "g" forces to the crewmembers, the rocket motor is provided with two concentric upper nozzles, secondary and auxiliary. The small auxiliary nozzle in the center of the upper nozzle fires simultaneously with the lower nozzle. This action provides 500 pounds of thrust to counteract slow-speed crew module pitch up at speeds below 300 knots. At speeds above 300 knots, after a .15-second delay, the upper nozzle burst diaphragm is severed by a flexible linear-shaped charge (FLSC) to increase the exhaust-flow area, thus increasing its thrust. Because of the increase in the exhaust-flow area, the rocket motor operating pressure is lowered, which results in reduced thrust of 9,000 pounds at the lower nozzle and increases the upper nozzle thrust to 7,000 pounds. This overall decreased thrust extends the operating time and reduces excessive "g" forces.
Dual-mode, q-actuated selector. In figure 5, you see the dual-mode, q-actuated selector. It continuously senses aircraft speed and selects the appropriate time delay. The letter "q" is used to identify forces or pressure required to actuate various pressure-sensitive aircraft devices. Pressure from the pitot static system, or ram pressure, is sensed at one end of the q-actuated selector, while dynamic pressure is sensed at the other end. Due to differential pressure, the q-actuated selector allows activation of a 1-second TDI to the barostat lock initiator and blocks propagation to the rocket motor upper nozzle when aircraft speed is less than 300 knots. When aircraft speed is greater than 300 knots, differential pressure is changed so that the q-actuated selector blocks propagation to the 1-second TDt and allows activation of SMDC to the. 15-second TDI to the rocket motor upper nozzle to fire.
4.4-second TDI. Another explosive train, with a 4.4-second time-delay initiator, is provided to back up both the dual-mode, qactuated selector and the g-sensor initiator. This equipment ensures that the barostat lock initiator is never activated more than 4.4 seconds after the rocket motor is fired.
1.0-second TDI. This time delay allows the module to gain altitude during a mode 1 ejection. Upon actuation, it fires the barostat lock initiator.
G-sensor initiator. The g-sensor initiator (fig. 6) is located in the survival equipment explosive device compartment. A TDI delays firing the g-sensor initiator for 1.6 seconds after rocket motor ignition during high-speed ejections. The forward motion of the crew module may be relatively high immediately after ejection of the crew module at speeds above 300 knots. After the forward motion decreases to approximately 2.2 (+/- 1 ) g' s, the g-sensor initiator fires and activates the barostat lock initiator.
Select/interrupt valve. The select/interrupt valve works similarly to the mechanical explosive interrupt. It either allows or blocks explosive propagation leading to the stabilization/brake parachute cutters. The direction of propagation depends on the mode of ejection selected by the dual-mode, q-actuated selector. When a mode 1 is selected, a detonation transfer assembly (DTA), which is another type of mild detonating cord, repositions the select/interrupt valve. When the recovery system operates, DTA propagation passes through the repositioned valve and fires the cutters.